Aviation Human Factors Training
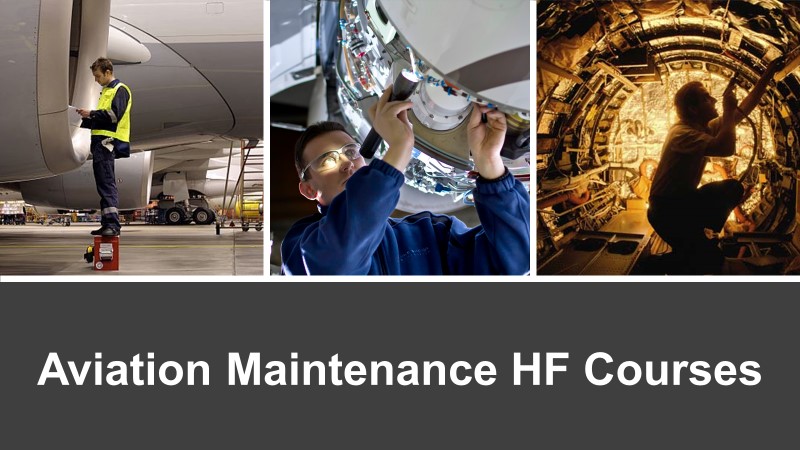
TACG is a worldwide leading provider of aviation Human Factors training. We have been providing Human Factors training to aviation operators on a worldwide basis since 2000. Our Human Factors courses are cost-effective, flexible, and practical (we minimize theory and maximize real-world experiences, examples, and case studies). TACG offers Human Factors courses for airline line maintenance facilities, MROs, FBOs, manufacturers, and more. TACG's Human Factors courses meet and exceed the requirements of Regulators worldwide (including FAA, Transport Canada, and EASA).